Biological Properties
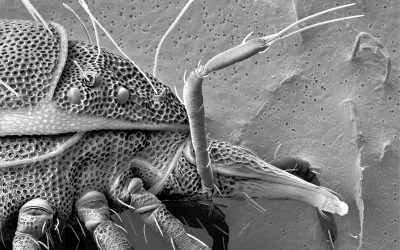
Microorganisms
Microorganisms are very small forms of life that can sometimes live as single cells, although many also form colonies of cells. A microscope is usually needed to see individual cells of these organisms.

Macro Fauna
While the most well‐known nematodes are pests occupying and feeding on plant roots (such as the lesion nematode and the soybean cyst nematode), in fact most nematodes are beneficial organisms. Nematodes are extremely important because they consume a diverse array of food sources, which places them at multiple trophic levels in the soil food web.

Particulate Organic Matter
Particulate organic matter (POM) fraction referred to in this document comprises all soil organic matter (SOM) particles less than 2 mm and greater than 0.053 mm in size (Cambardella and Elliot, 1992). POM is biologically and chemically active and is part of the labile (easily decomposable) pool of soil organic matter (SOM).
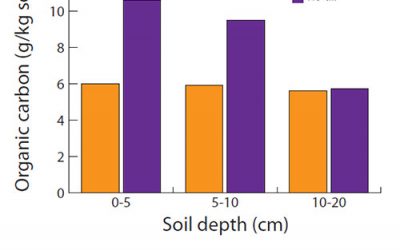
Total Organic Carbon
Total organic carbon (TOC) is the carbon (C) stored in soil organic matter (SOM). Organic carbon (OC) enters the soil through the decomposition of plant and animal residues, root exudates, living and dead microorganisms, and soil biota.
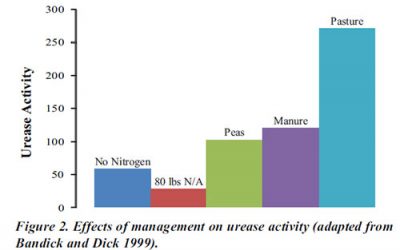
Soil Enzymes
Soil enzymes increase the reaction rate at which plant residues decompose and release plant available nutrients. The substance acted upon by a soil enzyme is called the substrate.
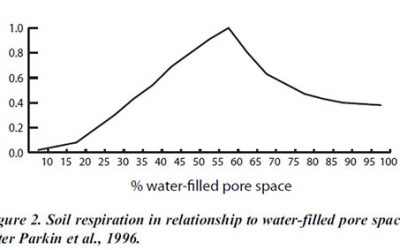
Soil Respiration
Carbon dioxide (CO2) release from the soil surface is referred to as soil respiration. This CO2 results from several sources, including aerobic microbial decomposition of soil organic matter (SOM) to obtain energy for their growth and functioning (microbial respiration), plant root and faunal respiration, and eventually from the dissolution of carbonates in soil solution.

West River Soil Health School Registration Open!
In 2024, the South Dakota Soil Health Coalition will host an additional Soil Health School in west of the Missouri River! The 2024 West River Soil Health School with be held June 26-27 near Caputa, SD! This school will focus on issues specific to the land, climate, and ag production systems of wester South Dakota. Class size is limited, so early registration is strongly encouraged!
News & Events
Farmer reaps higher yields by interseeding soybeans
By Stan Wise Alex Frasier has spent a lot of time studying what it takes to grow a successful crop. After studying ag production and precision technology at Lake Area Technical College, he has worked in ag retail and currently works as an agronomist in Aberdeen, SD....
Farm and ranch innovators to share new ideas at Soil Health Conference
By Stan Wise PIERRE, SD — Before Cooper Hibbard came home to manage his family’s ranch, he studied ag business, rangeland resources and Spanish at California Polytechnic State University and then worked on ranches all over the world. That education and experience...
Wintertime is decision time
By Stan Wise PIERRE, SD – It’s often said that the best time to start improving your land was 20 years ago, but the second-best time is right now. That statement might be harder for ranchers to swallow with winter on their doorstep, nothing growing in their pastures,...